Projects: A
Self-organization of small and medium-sized synthetic molecules through multiple interactions
A1: Systematic multidimensional quantification of multiple interaction patterns between polyphilic molecules
Supervisor: Prof. Daniel Sebastiani
Contact: daniel.sebastiani@chemie.uni-halle.de
The concept of “philicity” is based on the idea of a competition between intermolecular interactions of different nature. The conventional terms “hydrophilic”, “hydrophobic”, “lipophilic”, “fluorophilic” and further flavors within this scheme can be understood as systems (mixtures of molecules) with outward-pointing sidegroups of type A and B leading to either of the following situations:
1. “A is B-phobic” (e.g. benzene =A is hydrophobic, water = B): Then, the intermolecular interactions of type A–A and B–B are enthalpically favorable compared to the interactions A–B, A–B, and this enthalpic advantage is not overcompensated by the genuine entropic advantage of the random spatial arrangement.
2. “A is B-philic” (e.g. methanol =A is hydrophilic, water = B): Then, the enthalpic difference between the interaction configurations (A–A, B–B) versus (A–B, A–B) is smaller than the corresponding entropic advantage.
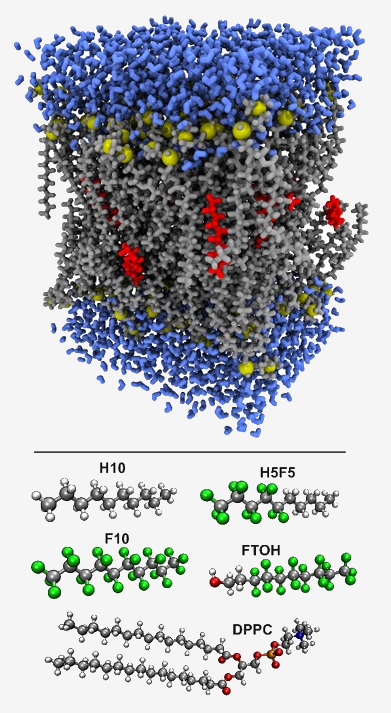
In this project the aim will be to derive a quantitative descriptor of philicity that includes all philicites, phobicities to have a theoretical description of multiple non-covalent interactions.
Main methods that will be used:
– Monte Carlo (MC) simulations
– Classical and quantum-mechanical molecular dynamics (MD) simulations
– Methods of quantum chemistry
A2 Experimental Part: Dynamic yet defined – self-assembly of small molecules in solution: colloid-like ionic clusters (ionoids)
Supervisor: Prof. Daniel Sebastiani & Prof. Dariush Hinderberger
Contact: daniel.sebastiani@chemie.uni-halle.de | dariush.hinderberger@chemie.uni-halle.de
We have recently discovered that small molecular building blocks with more than one interacting funtional group dynamically self assemble into non-trivial, non-random (thermal) structures in solution. This self-assembly into ionoids (as we termed them) takes place through a combination of preferential solvation, viscosity, van der Waals interactions together with long-range correlated electrostatics.
In this project in which two doctoral researchers will be supervised by Prof. Dr. Dariush Hinderberger and Prof. Dr. Daniel Sebastiani, we aim at deriving the full design principles that govern the formation of these dynamic structures from isotropic “clouds” of ions in solution through concepts of multiple non-covalent interactions.
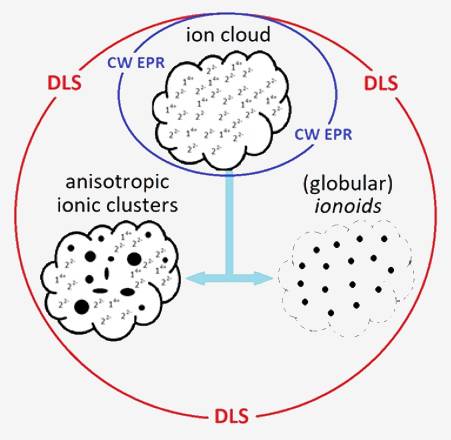
Experimental Physical-Chemical Project Main methods that will be used:
– Electron paramagnetic resonance (EPR) spectroscopy
– Dynamic light scattering (DLS) and Electrophoretic light scattering (ELS)
– Chemical synthesis of the ionoid building blocks
– Static light and potentially x-ray scattering (SLS, SAXS/WAXS)
– Other physico-chemical characterization methods (e.g. calorimetry)
– Mathematical (analytical) description of the ionoid-formation incubation process (e.g. based on bifurcation theory)
A3 Experimental Part: Design of liquid quasicrystals and their approximants
Supervisor: Prof. C. Tschierske
Contact: carsten.tschierske@chemie.uni-halle.de
Quasicrystalline packings have been detected in metal alloys by Nobel laureate D. Schechtmann. Meanwhile, quasicrystalline order was also found in block-copolymer morphologies, colloidal and nano-particle assemblies. Formation of quasiperiodic 2D nets is known in monomolecular layers on surfaces, which depend on the support by a surface lattice and the stabilization by surface interactions.
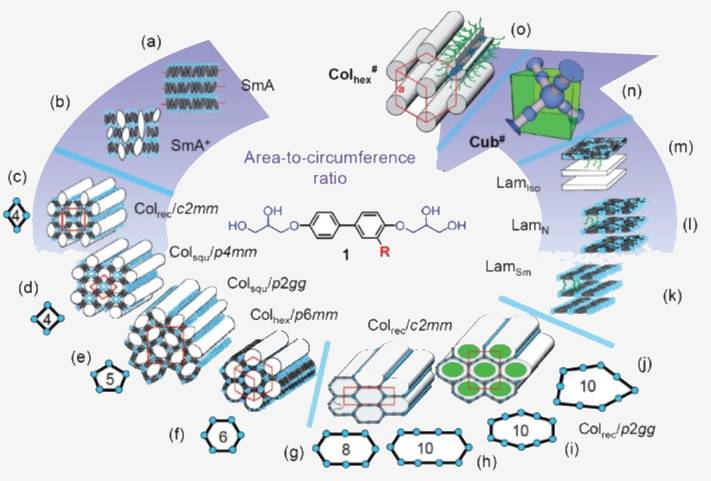
To date, liquid quasicrystals (LQC) have only been reported for 3D packings of spheres. In this project, in which two doctoral researchers will be supervised by Prof. Dr. Carsten Tschierske and Prof. Dr. Rebecca Waldecker, we aim at the design and understanding of new liquid quasicrystals with 2D packing of polygonal columns. This project involves the synthesis of new materials, their detailed investigations and mathematical analysis to discover new quasicrystalline packing modes and their periodic approximants in soft-matter structures, and to gain a full understanding of the molecular self-assembly in these liquid quasicrystals.
The experimental part of this project is supervised by Prof. C. Tschierske and will focus on:
– Organic and metallorganic synthesis of new polyphilic compounds and
– Their fundamental characterization by different physico-chemical characterization methods, like calorimetry, polarizing microscopy and X-ray scattering.
A3 Theoretical Part: Design of liquid quasicrystals and their approximants
Supervisor: Prof. R. Waldecker
Contact: rebecca.waldecker@mathematik.uni-halle.de
Quasicrystalline packings have been detected in metal alloys by Nobel laureate D. Schechtmann. Meanwhile, quasicrystalline order was also found in block-copolymer morphologies, colloidal and nano-particle assemblies. Formation of quasiperiodic 2D nets is known in monomolecular layers on surfaces, which depend on the support by a surface lattice and the stabilization by surface interactions.
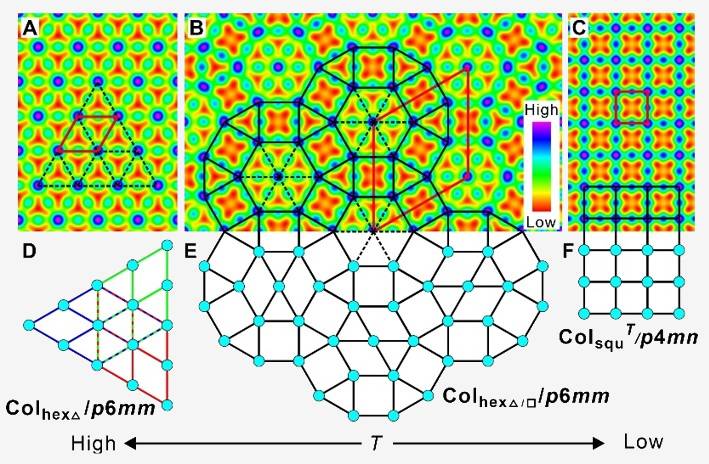
To date, liquid quasicrystals (LQC) have only been reported for 3D packings of spheres where the alternation of layers with different periodic sphere arrangements leads to the quasiperiodic order. In this project, in which two doctoral researchers will be supervised by Prof. Dr. Carsten Tschierske and Prof. Dr. Rebecca Waldecker, we aim at the design and understanding of new liquid quasicrystals with 2D packing of polygonal columns. In the theoretical part of this project, which is supervised by Rebecca Waldecker, we study mathematical aspects of the quasiperiodic tilings that occur.
– What tilings are most likely, given the molecular structure of the material in question?
– What happens if we introduce additional elements of geometric frustration in order to disable organisation on a periodic lattice?
– What quasiperiodic tiling patterns will appear instead?
Based on the existing literature on patterns and tilings we will analyse symmetry properties and develop a mathematical framework in which we can describe the initial self-organisation and then explain the changes that occur, for example the transition into a new, stable pattern when the circumstances change. Beginning by understanding the structures that have already been found and analysed in experiments, we aim for a prediction of new quasiperiodic soft-matter structures based on the mathematical theory.
A4: Characterizing the interaction of proteins with polyphilic detergents and lipids and their impact on membrane self-assembly
Supervisor: Jun.-Prof. Carla Schmidt
Contact: carla.schmidt@biochemtech.uni-halle.de
Biological membranes are formed by a phospholipid bilayer matrix and separate the aqueous interior of cells or cellular compartments from the mostly aqueous outer environment. This separation, however, makes the transport of material and information through the membrane necessary – a task which is often carried out by proteins that reside in the membranes and interact with the surrounding lipids. The notion that these interactions bear functional importance has recently received increasing attention. Natural protein-lipid interactions are diverse and include hydrophobic as well as polar interactions. To study these polyphilic protein-lipid interactions, we will follow a multi-disciplinary approach. We will mostly employ native mass spectrometry, which allows maintaining the non-covalent interactions between proteins and their ligands.
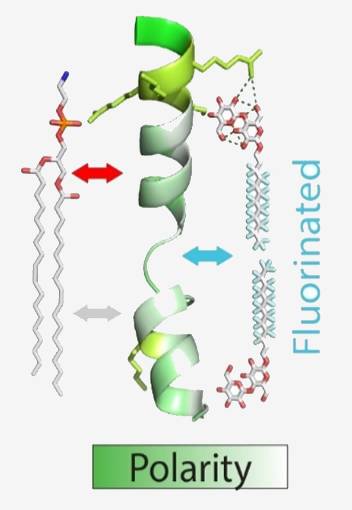
We will further combine native mass spectrometry with ion mobility to study unfolding of membrane proteins. For comparison, protein-membrane interactions will be characterised thermodynamically and spectroscopically. We will make use of different membrane mimetics with various lipid compositions to assess their impact onto protein binding and membrane insertion.
Main methods that will be used:
– Native mass spectrometry
– Ion mobility mass spectrometry
– Isothermal titration calorimetry (ITC) – Differential scanning calorimetry (DSC)
– Electron paramagnetic and nuclear magnetic resonance (EPR and NMR)
– Langmuir monolayer technique combined with infrared refection absorption spectroscopy (IRRAS)
A5: Studying amino acid interactions with non-natural polyphilic substrates
Supervisor: Jun.-Prof. M. Weissenborn
Contact: martin.weissenborn@ipb-halle.de
The interaction and binding of enzymes to substrates is not a static procedure as suggested by the simple key-lock concept, but a dynamic process repeatedly referred to as “induced fit” that often utilizes amphiphilic and more interactions.
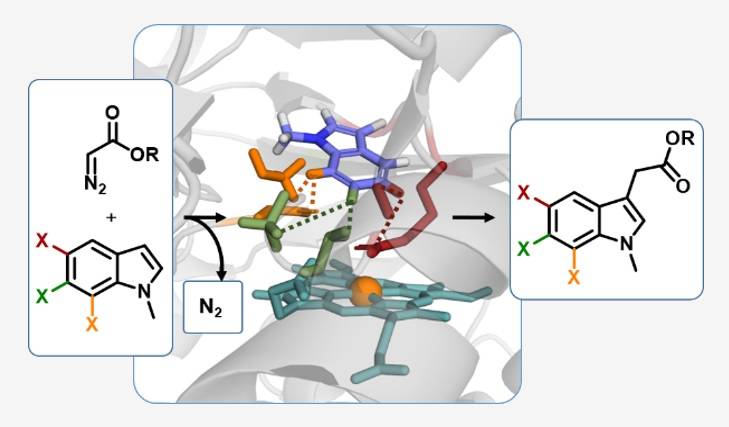
The different interactions between amino acids and substrates are able to precisely position the educt molecules in the active site of an enzyme allowing catalytic conversions in exceptional chemo-, regio- and stereoselectivities. This is due to the precise non-covalent positioning.
In this project, we aim to use this feature for controlled radical reactions in enzymes, which have not been seen in nature. Using directed evolution, the enzymes are taught to “use” the philicities of the substrate to allow these novel radical rearrangements.
A6: Covalent Organic Frameworks (COFs) beyond amphiphilic interactions.
Supervisor: Prof. Frederik Haase
Contact: frederik.haas@chemie.uni-halle.de
COFs are an emerging class of porous materials with high surface area and tunable pore sizes that have promising applications in gas storage, catalysis, and sensing. In this project, we aim to go beyond the traditional approach of tuning COFs’ hydrophobic and hydrophilic properties to explore other interactions that can be used to modify COFs’ structure and properties.
We will work on the design and synthesis of COFs with functional groups that can interact with other molecules beyond amphiphilic interactions. We use computational tools to design new COF structures with desired properties, and then synthesize them using organic synthesis techniques. We then characterize the resulting COFs using various techniques such as X-ray diffraction, Fourier transform infrared spectroscopy, and thermogravimetric analysis. We also evaluate their properties using gas adsorption measurements and solid state NMR. We will also work closely with other BEAM members to understand the fundamental principles that govern the behavior of COFs in various environments.
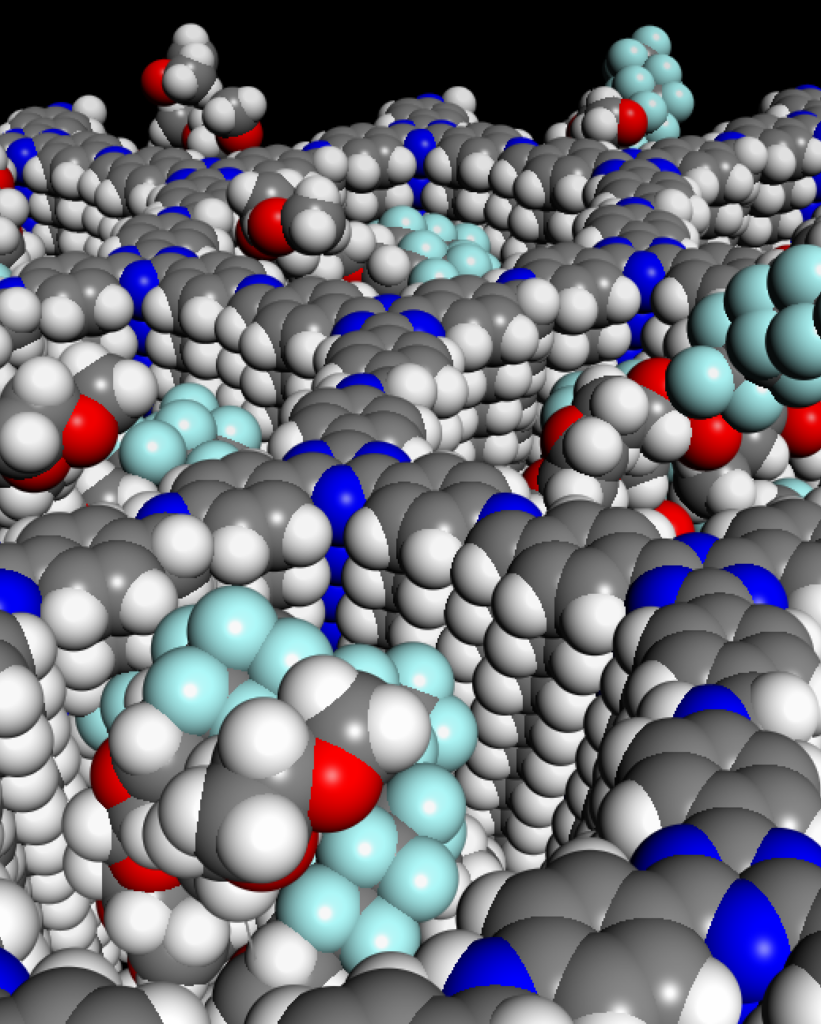